Search this Site
Culture, Climate Science & Education
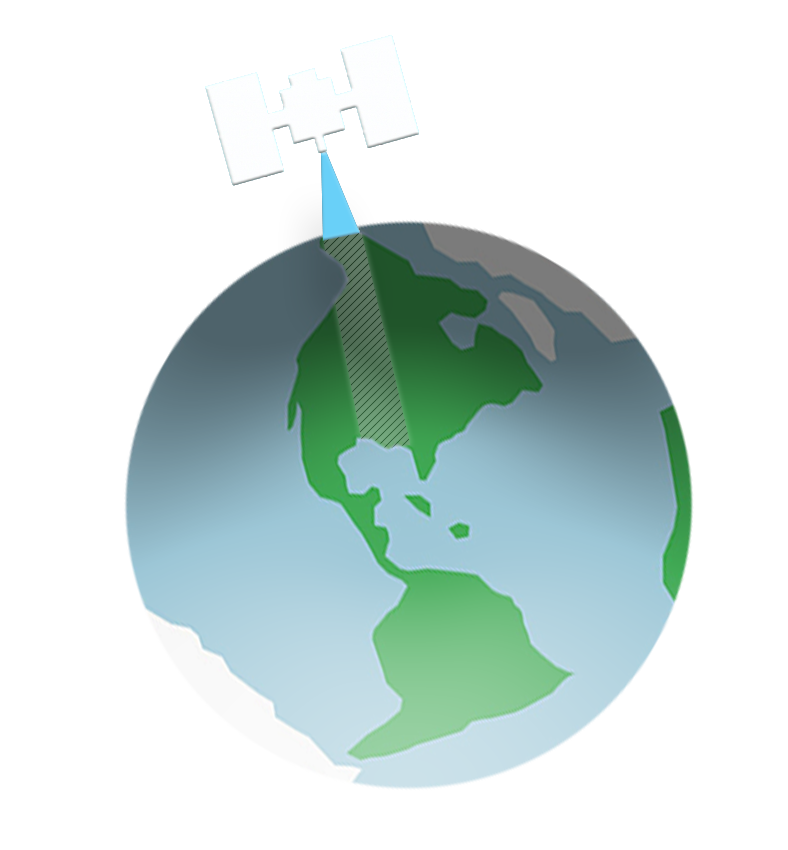
SECTION TWO
Remote Sensing Basics
Click the Bubbles Above or the Double Arrows () to Explore this Section
A. Evidence from Light
  Click this image to expand it.

Spectrogram of an acoustic recording from the western small-footed myotis (Myotis ciliolabrum). Ultrasonic microphones can record bat “chatter”, which is inaudible to human ears. Analysis of sound waves reveals bat species and population size. The recording is technically a remote sensing technique to detect echolocation—itself a bat remote sensing skill. At the Salish Kootenai College, instructor Janene Lichtenberg and Wildlife Biology student Sarah Twoteeth use this technique to monitor threatened bat species on the Flathead Indian Reservation. Credit: Ernest Valdez 2016, USGS. Public domain. Cited 2018 Dec 7. 
Broadly speaking, remote sensing is collecting information about a feature without direct contact. Your eyes are performing remote sensing right now, reading this sentence. Your ears gather evidence about your surroundings without you having to touch anything. Remote sensing uses energy—such as light or sound waves—to gather information.
Examples of remote sensing in the natural world include echolocation by bats and whales. Some blind people can similarly gain a sense of their surroundings with echoes from cane-taps or hard-soled shoes, as the musician Ray Charles is said to have done.
  Activity One
(Click to go to Activity One)
Activity One
Your eyes capture light for your brain to interpret. Look around you—the light you see first comes from a source (the sun, a light bulb), bounces off an object, and then enters your eye. Take a minute to write down some information you can gather about your surroundings just from seeing this reflected light. What visual clues did you use?
From my desk I can see two baskets. The color of light reflecting off one’s surfaces tells me it is natural reed and texture of the other basket seems to be a plastic-like material. I also see a green bean can fashioned into a homemade banjo—color tells me the can is steel and the string appears bronze, while the visual pattern of wood grain indicates the neck is oak. The cat, startled by cat-seeking children, bolts from the couch and behind a curtain—if my eyes could see other wavelengths of “light” energy (thermal infrared, as thermal goggles and some satellite sensors can) we could detect her hiding place.
Light energy reflected from a surface holds information about what a feature is and from what material it is made.
Click the Bubbles Above or the Double Arrows () to Explore this Principle
B. Remote Sensing
  Click this image to expand it.

From: https://science.nasa.gov/earth-science
In common usage, the term “remote sensing” usually refers to the collection and analysis of imagery from satellites or aircraft, used to observe and map features on the Earth’s surface or atmosphere. Earth observations and analysis of these images will be our focus throughout the course.
The illustration at left (click it to enlarge it) shows current and planned NASA Earth observing satellites. These satellites have different missions (e.g. monitoring vegetation, detecting glacial retreat) and gather different kinds of light energy (i.e. information) to accomplish their purpose.
For example, Landsat satellites can “see” visible and infrared light—among other applications, climate scientists use this to map the timing of spring green-up. The satellite called SMAP detects a different “light” energy—radio and microwaves—for measuring soil moisture from space.
  Activity Two
(Click to go to Activity Two)
Activity Two
Ask your parents or grandparents or an elder if they’ve noticed changes in the seasons, like spring arriving earlier or later since childhood.
Next you will learn basic properties of light energy and how we can gather information from it.
  In the News
(Click to Read)
PENGUIN POOP, SEEN FROM SPACE, TELLS OUR CLIMATE STORY
Source: https://www.wired.com/story/penguin-poop-antarctica-climate-change/
NICK GARBUTT/BARCROFT/GETTY IMAGES
SATELLITES WATCH MANY things as they orbit the Earth: hurricanes brewing in the Caribbean, tropical forests burning in the Amazon, even North Korean soldiers building missile launchers. But some researchers have found a new way to use satellites to figure out what penguins eat by capturing images of the animal’s poop deposits across Antarctica.
A group of scientists studying Adélie penguins and climate change have found that the color of penguin droppings indicates whether the animals ate shrimp-like krill (reddish orange) or silverfish (blue). The distinction is interesting because the penguin's diet serves as an indicator of the response of the marine ecosystem to climate change. Separate research is starting to show, for example, that penguin chicks that are forced to rely on krill as their main source of food don't grow as much as those who have fish in their diet.
The penguins' guano deposits build up over time on the rocky outcroppings where the birds congregate, making them colorful landmarks. The researchers took samples from the penguin colonies, found their spectral wavelength, then matched this color to images taken from the orbiting Landsat-7 satellite.
“There’s a clear regional difference, krill on the west, fish on the east,” says Casey Youngflesh, a postdoctoral researcher at the University of Connecticut who presented his findings last week at the annual meeting of the American Geophysical Union in Washington. It's the first time that scientists have been able to track diet from space, and researchers say it's a new tool for looking at how certain seabird and penguin populations are doing on other regions of the planet.
Knowing what, and how much, five million breeding pairs of Adélie penguins are eating is important because it tells researchers how the base of the food chain is doing. The population of tiny krill appears to be crashing on the western side of the Antarctic peninsula, the 800-mile thumb that sticks up toward the tip of South America. Rapidly warming, changing climactic conditions as well as a huge increase in industrial-scale fishing, have taken a toll on these small crustaceans.
Krill are harvested commercially for use in pet food and nutritional supplements, but for many penguins, it’s the basis of their diet. As krill have become more scarce, so, too, have the penguins in western Antarctica who like to eat them. “Diet can tell us how food webs are shifting over time,” says Youngflesh. “It would take a lot of time and a lot of money to visit all these sites. Climate change is extremely complicated and we need data on large scales.”
Youngflesh says he hopes the color-coded poop maps can be used to track penguin populations in the future, as well as other seabirds across the globe. That’s because seabirds aggregate in the same places as penguins and eat the same things. Of course, this form of remote sensing can’t tell researchers how penguins’ diets compare across time. So one researcher dug through the guano itself in search of insights into the penguins' history.
“There are unanswered questions about when did they arrive, how have their diets changed over time,” says Michael Polito, assistant professor of oceanography and coastal sciences at Louisiana State University. “Those are questions satellites can’t answer, and it was my job to dig it up.”
THOMAS SAYRE-MCCORD/WHOI/MIT
Polito excavated mounds of guano, feathers, bones and eggshells on the remote Danger Islands, a large penguin colony on the tip of the Antarctic Peninsula that has remained mostly free of human visitors. When he reached the bottom of the pile, he took the material back to his lab and applied radiocarbon techniques to figure out the age of the first penguin settlers. He found that the penguins have been living on Danger Islands for nearly 3,000 years. Since Adélie penguins need access to ice-free land, open water and a plentiful food supply to feed their baby chicks, the presence or absence of a penguin colony is a sign of the climate conditions at the time, Polito says. Polito's new study pushes back the time of penguin's arrival there by 2,200 years for that region and confirms other data taken from ice cores and sediments about the history of that region's climate.
"This ability to estimate penguin diets from space will be a real game changer for science in Antarctica," Polito said. "It really takes a lot of time and effort to figure out what penguins eat using traditional methods so being able to evaluate diets all around the Antarctic continent from space is a pretty amazing leap forward."
The combination of digging through poop and analyzing images from satellites is giving researchers a better handle on possible trouble spots for the Adélie penguin, as well as its cousins the chinstrap, Gentoo and emperor penguins. The laboratory of Heather Lynch, associate professor of ecology and evolution at Stony Brook University, put together a nifty continent-wide map of penguin colonies from the four species, and is using citizen volunteers to count them one by one. Lynch's group is also beginning to look back at previous satellite images taken from the 1980s until now to see if they can establish the same penguin poop-diet connection.
Click the Bubbles Above or the Double Arrows () to Explore this Principle
C. Light is Electromagnetic Energy
  Click this image to expand it.
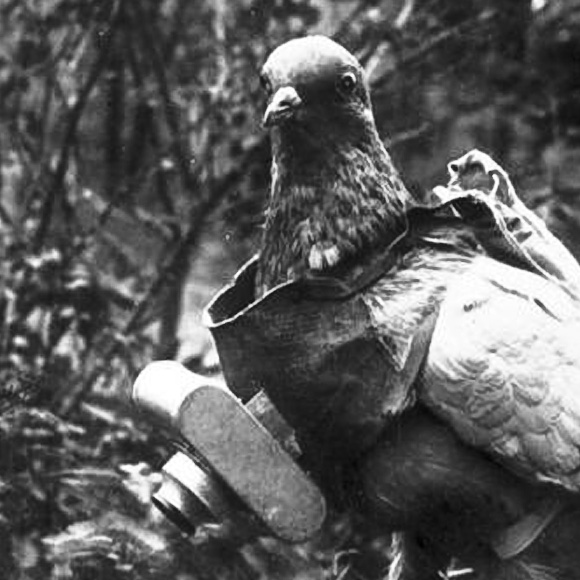
Tour of the Electromagnetic Spectrum. From: NASA. [December 06, 2018, cited 2018 Dec 7]. https://science.nasa.gov/ems/
The primary form of energy detected with remote sensing is electromagnetic energy. Visible light is one kind of electromagnetic energy. X-rays, ultraviolet light, infrared radiation, and radio waves are other kinds of electromagnetic energy.
Light energy behaves in a wave-like fashion (similar to sound or ocean waves in many respects) and visible light of different wavelengths appears to us as different colors. The shortest wavelength humans can see is blue-violet (~400-nanometers) and the longest wavelength is red (~700-nm). The light visible to human eyes is a small portion of the larger electromagnetic spectrum (EMS).
Imaging sensors record light reflected from a surface or object. We can measure the intensity of light from specific regions of the EMS (e.g. green, or infrared), which can reveal the physical and chemical composition of the object.
This ability of sensors to measure light reflectance in specific wavelengths—in addition to their ability to detect “invisible” wavelengths—makes remotes sensing a very powerful tool. Remote sensing reveals a wealth of information beyond what the human eye can see. (Click the image above to enlarge it.)

Animal Vision
Animal Vision
Other animals likely have differing color perception and vision compared to humans. Mantis shrimp have sensitivity to a wider range (300 nm – 720 nm) of light wavelengths, well into the ultraviolet (UV) and near infrared regions (Thorn et al 2014). Many insects, especially bees, are though to see into the UV range, possibly to aid in flower detection. The kinds and layout of color-sensitive pigments in an eye gives a clue as to what the viewer perceives. Why would various species have different perceptions of their environment?
  Click this image to expand it.

Source: https://www.cyberphysics.co.uk/
Terminology: light and the electromagnetic spectrum
Some scientists use the term “light” as shorthand for all electromagnetic energy—acknowledging all wavelengths are variations of the same phenomenon. “Ultraviolet light” and “infrared light” are commonly used terms and neither is visible. Others prefer to always specify “visible light” from other kinds of electromagnetic (EM) energy. This course will sometimes use “light” to refer to any energy in the electromagnetic spectrum (EMS). Energy in the EMS can also be referred to as “radiation”.
People divide the EMS into regions of wavelengths with similar properties (e.g. visible light, infrared), but there are no sharp divisions. Properties change gradually over the spectrum.
You may notice from the graphic at right (click it to enlarge it) that wavelength and frequency are related; EM travels at 299,792,458 meters per second, the speed of light. Different scientific disciplines use wavelength, frequency, or energy to describe categories of electromagnetic energy—frequency is the norm for radio studies while wavelength is common in the remote sensing community.
Wavelengths used in remote sensing are commonly expressed in either micrometers (μm; 0.000001 m or 10-6 m) or nanometers (nm; 10-9 m). The longest wavelength red light can be described as either 700 μm or 0.7 nm.
  Activity Three
(Click to go to Activity Three)
Activity Three
Wavelengths of visible light are difficult to imagine—550-nanometers (nm) is a shade of green—but radio waves are in a scale familiar to people—meters. Figure out the wavelength of you favorite radio station. FM radio stations are measured in megahertz. Example: turning the dial to 98.5 finds a frequency of 98.5 x 106 cycles/second or 98,500,000/s. The speed of light is about 3.0×108 m/s, or specifically 299,792,458 m/s.
Wavelength = speed/frequency Symbolized as: λ = c/f
Wavelength of FM station 98.5 = 3.044 meters
  In the News
(Click to Read)
What Two Colors Combine To Make Blue?
by DANIEL NELSON NOVEMBER 29, 2018
Source: https://sciencetrends.com/what-two-colors-combine-to-make-blue/
What two colors combine together to make the color blue? The answer to this question depends on whether you are talking about the blue which is part of the visible light spectrum, or certain pigments of materials that can be mixed together.
In terms of the light spectrum blue is a primary color, which means that it is one of the base colors that exists in the universe and it cannot be created by combining different colors together. The other primary colors of light are green and red. This is why when using digital paint programs, the values are given in RGB (Red, Green, Blue) format.
Photo: Aquilatin via Pixabay, CC0
When talking about combining pigments together, such as when painting, the calculation is slightly different. Pigments don’t emit or produce light of a certain color, rather they get their colors by absorbing specific wavelengths of color. The primary pigment colors are cyan, magenta, and yellow. Cyan absorbs red, yellow absorbs blue, and magenta absorbs green. Therefore, in order to get a blue coloration from pigments you would need to absorb the red and green light colors, which can be achieved by mixing magenta and cyan.
The Light Spectrum
Photo: D-Kuru via Wikimedia Commons, CC 3.0, https://en.wikipedia.org/wiki/Visible_spectrum#/media/File:Light_dispersion_of_a_mercury-vapor_lamp_with_a_flint_glass_prism_IPNr%C2%B00125.jpg
Electromagnetic radiation is everywhere in the entire universe. Electromagnetic radiation comes in different forms such as radio waves, ultraviolet radiation, infrared radiation, microwaves, and visible light. What we define as visible light is just the wavelengths of electromagnetic radiation that are visible to the human eyes.
Electromagnetic radiation is transmitted by particles or waves at various frequencies and wavelengths, and the range of wavelengths that radiation can be transmitted in is referred to as the electromagnetic spectrum. The electromagnetic (or EM) spectrum is typically divided into seven different regions. These regions are listed in order of increasing energy frequency and decreasing wavelength. The most common labels for these seven regions are as follows: radio waves, microwaves, infrared, visible light, ultraviolet, x-rays, and gamma rays.
Representation of the colors at their respective wavelengths in the light spectrum. By Gringer – Own work, Public Domain, https://commons.wikimedia.org/w/index.php?curid=4639774
Visible light is the light that falls on the electromagnetic spectrum between infrared wavelengths and ultraviolet wavelengths, and the frequency of visible light is approximately 4×10^14 to 8 x 10^14 cycles every second. This can also be expressed as hertz in wavelengths of approximately 740 nm to 380 nm.
Color and temperature are correlated with one another. This is because when objects grow hotter the energy the object radiates is primarily made up of shorter wavelengths, and this looks like the changing of colors to the human eye. An easy example to visualize is a blowtorch. Initially the flame of a blowtorch is reddish, yet it turns bluish as the flame is adjusted and becomes hotter. Incandescence is the process of converting heat energy into light energy.
Incandescent light is emitted when hot material emits photons, part of the material’s thermal vibration energy. The energy radiated by a hot object reaches the infrared portion of the EM spectrum at approximately 800°C or 1470° Fahrenheit. As the temperature climbs beyond this point, the radiated energy climbs into the visible portion of the EM spectrum, and the object will begin to glow red. If the object continues to get hotter still it will gain a white-hot color, and then a blue color after that.
As you may be able to guess, because color and temperature are related, astronomers are able to look at interstellar objects like stars and estimate their temperatures based off of their color. For instance, the light emitted by the sun has a wavelength of around 550 NM, which is perceived as either yellow or whitish light. This is the result of the surface of the sun being around 5800° Kelvin, or 5527°C or 9980° F. Cooler stars, those around 3000°C or so, would give off a more reddish glow, such as the star Betelgeuse. Meanwhile, hotter stars, such as those with a Temperature of around 12,000°C would give off a bluish light, like Rigel.
The wavelengths of light an object gives off can also help astronomers make inferences about the composition of an object. This is because every element absorbs different wavelengths of light, something referred to as the absorption spectrum. By analyzing what part of the spectrum is being absorbed by objects, astronomers can determine the likely chemical composition of things like asteroids, dust clouds, and stars.
The most salient aspect of the visible light spectrum for humans is color. Color is both a result of the way the human eye perceives light and an inherent property of the light itself. Objects don’t actually possess color, rather they refract or give off light which is in a certain wavelength and therefore appears to be a certain color.
The Perception Of Light
Drawing of a cone cell. Photo: By Ivo Kruusamägi – Own work, CC BY-SA 3.0, https://commons.wikimedia.org/w/index.php?curid=54680466
The human eye has a variety of specialized light receptor cells, referred to as rods and cones. Rods are cells that are specialized for vision at low light levels, taking in the visible light and sending it to the brain for processing. Meanwhile, cones are receivers that deal with the wavelengths of the EM spectrum which correspond with color. Wavelengths of light at the lower end of the visible spectrum (those that are around 740 NM) are interpreted as red, while light in the middle portion of the visible spectrum is seen as green, and wavelengths of light at the upper end of the spectrum (those around 380 NM) are seen as violet. The other colors that the human eye perceives are simply mixtures of these three different colors.
The color yellow for instance, is made up of both green and red while cyan is a mixture of blue and green, and magenta is a mixture of blue and red. White light occurs when all the colors are combined together, while black is the absence of light. The fact that white light is made up of the three colors on the visible spectrum was realized by Isaac Newton, who passed some light through a prism and found that there was a color spectrum projected onto a nearby wall. As for the color blue, the true blue of the primary color is witnessed at around a wavelength of 470.
How Pigments Work
Pigments work not by adding light together, but by removing some of the light frequencies from the combination of frequencies that creates a white light. With pigments, the colors that are absorbed by the pigment are the colors you won’t see, you will only see the colors that bounce off the object and enter your eye. This system of coloration is called subtractive color, and it is what is used in dyes and paints. The molecules within the paint or dye absorb certain frequencies and reflect the other frequencies away, and these reflected frequencies are what the brain interprets as the color of that object.
For instance, a famous pigment is known as chlorophyll, and this pigment absorbs the red and blue parts of the visible spectrum, reflecting the green light. Chlorophyll is what gives green plants their coloration.
Other examples of biological pigments include:
- Hemoglobin, which is responsible for giving red blood cells their red color.
- Carotenoids are pigments produced by certain bacterias, among these is carotene which gives carrots and flamingos their orange/pink coloration.
- Anthocyanins, a type of water-soluble pigment that is found in the tissues of higher plants and can appear as red or blue.
- Melanin, a type of pigment found in most organisms which has a role in shielding cells from ultraviolet radiation.
- Poyene enolates, a specific type of red pigment unique to certain species of parrots.
The atomic structure of an object determines what color it absorbs. The vibration frequency of the electrons in the object will be at or near the frequency of the incoming light waves. Because of this, the electrons in the material will absorb the light energy and begin to vibrate. If the atoms within the material hold onto their electrons tightly, the vibrations will be passed along the chain to the nuclei of the atoms. As a result, the atoms will speed up and hit into other atoms, giving off the heat energy that was acquired during the vibration process.
As mentioned, when mixing pigments together, blue can be made by mixing cyan and magenta together.
Hues And Shades Of Blue
When you have a standard blue pigment, you can get other shades and hues of blues by combining other shades, hues, or colors together with it. For instance, if you want a lighter blue, you can combine aquamarine and white. Meanwhile, a royal shade of blue can be made by combining aquamarine and pink. A grayish blue can be made by combining brown and blue, while a dark blue can be made by combining standard/aquamarine blue and black.
The Electromagnetic Spectrum: A Summary

-
Radio
Radio Waves
- Radio waves have the longest wavelengths in the Electromagnetic spectrum, ranging from about 0.04 inches to more than 62 miles.They also have the lowest frequencies, from about 3,000 cycles per second or 3 kilohertz (kHz) up to about 300 billion hertz, or 300 gigahertz (GHz).
- The best-known use of radio waves is for communication; television, cellphones and radios all receive radio waves and convert them to mechanical vibrations in the speaker to create sound waves that can be heard.
- Radio waves have the longest wavelengths in the Electromagnetic spectrum, ranging from about 0.04 inches to more than 62 miles.They also have the lowest frequencies, from about 3,000 cycles per second or 3 kilohertz (kHz) up to about 300 billion hertz, or 300 gigahertz (GHz).
-
Microwave
Microwaves
- Microwaves have wavelengths of about 12 inches to 1 to 0.04 inches.They have frequencies ranging from about 1 billion cycles per second, or 1 gigahertz (GHz), up to about 300 gigahertz.
- This region is further divided into a number of bands, with designations such as L, S, C, X and K.
-
Infrared
Infrared
- Infrared waves are longer than those of visible light, just beyond the red end of the visible spectrum. It has wavelengths of about 12 inches to 0.00003 inches and frequencies from about 3 GHz up to about 400 THz, although these values are not definitive.
- Infrared (IR) falls in the range of the (EM) spectrum between microwaves and visible light.
-
Visible
Visible
- Visible light has wavelengths of about 740 nanometers (nm) or 2.9 × 10−5 inches, to 380 nm (1.5 × 10−5 inches). It has frequencies of about 4 × 1014 to 8 × 1014 cycles per second, or hertz (Hz)
- It falls in the range of the EM spectrum between infrared (IR) and ultraviolet (UV).
-
Ultraviolet
Ultraviolet
- Ultraviolet (UV) light has wavelengths of about 380 nanometers (1.5 × 10−5 inches) to about 10 nm (4 × 10−7 inches). It has frequencies of about 8 × 1014 to 3 × 1016 cycles per second, or hertz (Hz).
- Ultraviolet (UV) light falls in the range of the EM spectrum between visible light and X-rays.
-
X-ray
X-ray     
- X-rays are roughly classified into soft X-rays and hard X-rays. Soft X-rays have relatively short wavelengths of about 10 nanometers (a nanometer is one-billionth of a meter). They fall in the range of the electromagnetic (EM) spectrum between ultraviolet (UV) light and gamma-rays.
- Hard X-rays have wavelengths of about 100 picometers (a picometer is one-trillionth of a meter).
- These electromagnetic waves occupy the same region of the EM spectrum as gamma-rays.
-
Gamma Ray
Gamma Rays
- Gamma-rays have wavelengths of less than 100 picometers (pm), or 4 x 10^9 inches (a picometer is one-trillionth of a meter). They have frequencies greater than about 1,018 cycles per second, or hertz (Hz).
- Gamma-rays fall in the range of the EM spectrum above soft X-rays. .
Click the Bubbles Above or the Double Arrows () to Explore this Principle
D. How Light Behaves
  Click this image to expand it.
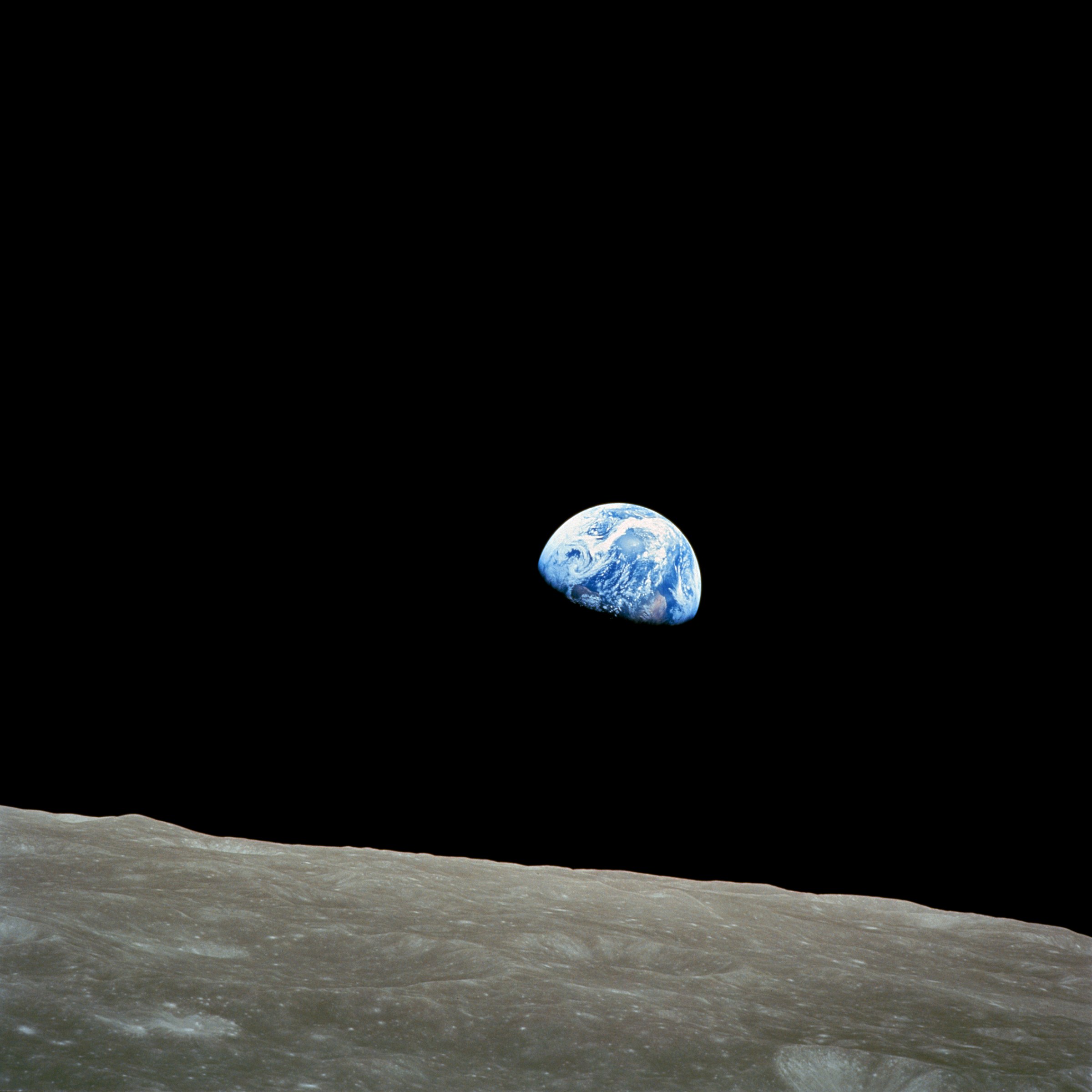
Light absorbed by several photosynthetic pigments in a spinach leaf. No pigments absorb green light—green is reflected—hence we perceive most leaves as green. Source: Emily Podlipski. 2013 March 6. Cited 2018 Dec 11. https://sbi4u2013.wordpress.com
/2013/03/06/chlorophyll-not-so-borophyll/
When electromagnetic radiation strikes an object (a leaf for example), it can be reflected, absorbed, or transmitted, i.e. passed through.
A portion of sunlight falling on a leaf is absorbed, transferring energy to photosynthetic pigments and other molecules in the leaf’s structure. Energy not captured by photosynthesis is emitted as heat.
A portion of sunlight on a leaf is reflected—if captured by our eye or camera an image is created. Note the graphic of reflectance (below) is reverse of absorbance. Where are the vegetation types and their reflectance curves most different? If we could see near infrared wavelengths, as is possible with remote sensing, the vegetation types would contrast well. In the visible light range (0.4 – 0.7 micrometers), the curves are more similar and visually, more difficult to tell apart.
  Click images to expand.

Emitted electromagnetic energy & thermal infrared. Source: Smith 2012. www.microimages.com

Source: Steeven
When electromagnetic radiation strikes an object (a leaf for example), it can be reflected, absorbed, or transmitted, i.e. passed through.
A portion of sunlight falling on a leaf is absorbed, transferring energy to photosynthetic pigments and other molecules in the leaf’s structure. Energy not captured by photosynthesis is emitted as heat.
A portion of sunlight on a leaf is reflected—if captured by our eye or camera an image is created. Note the graphic of reflectance (below) is reverse of absorbance. Where are the vegetation types and their reflectance curves most different? If we could see near infrared wavelengths, as is possible with remote sensing, the vegetation types would contrast well. In the visible light range (0.4 – 0.7 micrometers), the curves are more similar and visually, more difficult to tell apart.
In addition to reflecting light, objects emit electromagnetic energy just due to their temperature (or more correctly, heat energy). For example, the human body at 98.6°F emits radiation at about 9.3 μm —in the invisible “long wave” infrared region. Hotter objects emit light of higher energy and smaller wavelength. Objects over ~1,160°F begin to glow red in the visible spectrum. The sun’s surface at 5,778 degrees Kelvin (just under 10,000°F) emits the most radiation at ~0.5 μm—visible green light. The sun also emits other colors, making sunlight white (below).
  Click this image to expand it.

Source: NASA. Cited 2018 Dec 10. https://mynasadata.larc.nasa.gov/
Do you find it interesting that animal eyes and plant leaves evolved pigments that use the most abundant light energy from the sun? Analyze the Solar Radiation graph; short wave infrared light from our sun is only 1/3 the intensity of visible light. If eyes evolved to see radiation around 1.6 μm wavelength, the world would appear three times as dark. Or maybe our eyes would be three times as large!
The kinds of wavelength emitted from objects at natural earth temperatures are called the thermal infrared range—the EMS region of ~3 to 14 μm. The Landsat 7 satellite’s thermal imager, used to measure earth surface temperature from space, measures wavelengths of 10.4 – 12.5 μm.
  Click this image to expand it.

Thermal and normal color images. Thermal infrared wavelengths pass through thin material like this plastic bag. However look at this man’s glasses—“visible” light transmits through glass but what about thermal infrared wavelengths? Source: NASA. 2006 Sept. [Accessed 2018 Dec11] http://coolcosmos.ipac.caltech.edu/cosmic_kids/learn_ir/index.html
Night Vision vs. Thermal Imaging
Night vision and thermal imaging devices—used by search & rescue and military—illustrate the difference between reflected and emitted light energy. Night vision amplifies dim “visible” light along with some near infrared, 0.4 to 1.0 μm, reflected from objects. No emitted (thermal infrared) radiation is used here—objects would have to be nearly 1,000°F to emit wavelengths detectable with night vision. Night vision will not allow you to see through smoke, curtains, etc.—you can’t do that with the visible spectrum in the first place. Also, if no visible light exists to amplify (e.g. completely dark room), your night vision display will be completely dark too.
Thermal imagers on the other hand display thermal infrared energy (3 to 12 μm) emitted from normal earth-temperature objects. Thermal infrared wavelengths penetrate smoke; the devices can operate in complete darkness and detect slight variations in temperature.

Click the Stork to Learn about Where Light Comes From
Where Does Light Come From?
Light is all around us and easy to take for granted, yet mysterious. So, where does light—and other electromagnetic energy—come from?
Light comes from excited matter. Matter can be excited by heat energy—in fact all matter with a temperature above absolute zero is excited—this causes an atom’s electron will jump up an orbital level. An electron will fall back to a more “stable state” (at a less energized orbit) and when it does energy is released in the form of a photon. Electron orbits occur at predictable, set, energy levels, so the released photon will have corresponding, set, energy and wavelength. This is why photons carry so much information about the matter they were emitted from. Atoms in the sun’s hot surface for example are always bumping into each other, gaining and loosing orbital states, and emitting radiation.
In a similar way, only certain levels—quanta—of energy can be absorbed by an atom (to excite an atom’s orbital level). Energy not matching will be reflected or transmitted.
Click the Bubbles Above or the Double Arrows () to Explore this Principle
E. Electromagnetic Radiation and the Atmosphere
Just as electromagnetic (EM) energy interacts with the earth’s surface, it is also absorbed, reflected, transmitted (and also scattered) by the atmosphere. Some wavelengths pass through nearly unobstructed, while others are completely blocked and never reach the earth’s surface.
Understanding this interaction is important. Light from the sun is the most common energy used in remote sensing—EM radiation must pass through the atmosphere, reflect off an earth surface, and travel through atmosphere again before it is observed by a satellite or aircraft sensor.
Atmospheric Absorption
The graphic shows regions of the electromagnetic spectrum (EMS) that transmit sunlight relatively unaffected. These “atmospheric windows” allow remote sensing to occur. The most used windows for remote sensing are the ultraviolet to infrared wavelengths (0.3 μm – 1.2 μm; this includes visible light), two mid-infrared windows (3 μm – 5 μm and 8 μm – 14 μm), and the microwave region. Shaded areas represent incoming wavelengths that are absorbed by gasses—the most significant are water vapor, carbon dioxide, and ozone.
Relating Back to Climate Change
Atmospheric absorption also relates directly back to climate change—this is part of the greenhouse effect warming earth’s atmosphere. What have you learned about EMS and energy absorption related to this?
In a nutshell, the atmosphere “lets in” shorter wavelength energy from the sun, especially through the UV-visible-infrared window. When this energy strikes the earth or the atmosphere, it is partially absorbed and reemitted at longer infrared wavelengths. This energy then gets trapped. What would prevent longer wavelengths of infrared energy from passing away from earth? Look at the previous graphic for specific answers.
Click the Bubbles Above or the Double Arrows () to Explore this Principle
Click the Bubbles Above or the Double Arrows () to Explore this Principle
G. References
Butcher G, Mottar J, Parkinson CL, Wollack EJ. 2016. Tour of the Electromagnetic Spectrum. 3rd ed. Washington DC, NASA. 32p. Available from: https://science.nasa.gov/ems/ ISBN 978-0-9967780-2-2
Tour of the Electromagnetic Spectrum [Internet]. NASA. [December 06, 2018, cited 2018 Dec 7]. https://science.nasa.gov/ems/
Le Treut H.; Somerville R.; Cubasch U.; Ding Y.; Mauritzen C.; Mokssit A.; Peterson T.; Prather M. Historical overview of climate change science (PDF). Retrieved 2018 October 14. in IPCC AR4 WG1 2007
Mantis shrimp can see UV-NIR (300-720 nm)
Thorn, Hanne H.; How, Martin J.; Chiou, Tsyr-Huei; Marshall, Nicholas Justin (January 24, 2014). "A Different Form of Color Vision in Mantis Shrimp". Science. 334 (6169): 411–413. Bibcode:2014Sci...343..411T. doi:10.1126/science.1245824.
"NASA GISS: Science Briefs: Greenhouse Gases: Refining the Role of Carbon Dioxide". www.giss.nasa.gov. Retrieved 2018 October 14.